Why Capturing Carbon from the Air Will Always Be Expensive
Don't Hold Your Breath for Large Negative Emissions
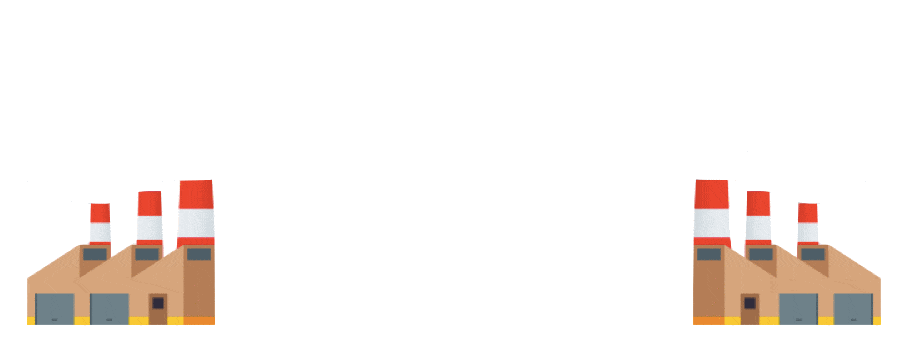
If you’ve been following the climate crisis, you’ve probably heard that we need to get to zero carbon emissions. Quite simply: the more CO₂ there is in the air, the more the planet warms, and so the only way to stop the warming is to stop emitting carbon.
This is a difficult task. It’s so difficult that many experts believe we won’t get to zero without some form of ‘negative carbon emissions’, and one big idea here is to develop new technologies that suck carbon dioxide out of the air on a global scale.
But any future technology extracting carbon from the air will never be a substitute for reducing our carbon emissions. That’s because there’s a basic cost to capturing carbon dioxide — a cost imposed by the laws of physics — and the bad news is, it isn’t cheap.
Why Extracting CO₂ From The Air is Inherently Inefficient
Let’s say you come across some advanced future technology that sucks CO₂ molecules out of the air (perhaps handed to you by an environmentally conscious time-traveler).
Surprisingly, even if we know nothing about how this machine works, we can still use physics to work out the minimum amount of energy that it consumes. All we need to know is what the machine does, and what it does is trap CO₂ molecules in a compartment (like a vacuum cleaner, but for CO₂).
Here’s a cartoon of what the air in your room looks like before you turn on the machine (the red dots represent CO₂ molecules).
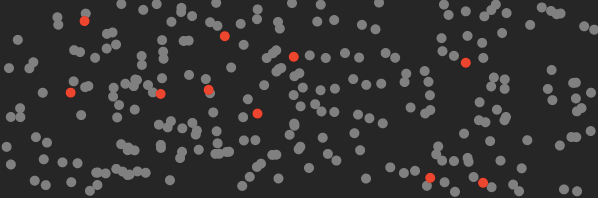
And here’s what it looks like after this machine traps the CO₂.
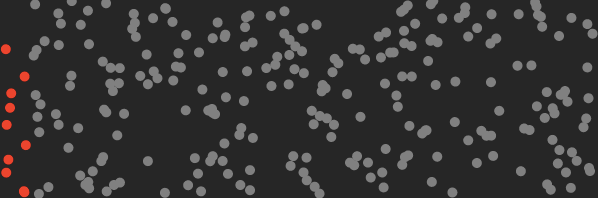
By separating CO₂ molecules, the machine reduces the entropy of the air by an amount that can be calculated.
Why Does This Machine Decrease The Air’s Entropy?
Roughly speaking, entropy measures the disorder of the air molecules. By separating the CO₂ we’ve arranged the molecules in a more orderly state, i.e. we’ve decreased its entropy.
You might think this entropy change is impossible to calculate without knowing more about the machine. But the air’s change in entropy depends on what happened, not how it happened. And since we know the initial and final state of the air — mixed vs. separated — we can calculate its change in entropy.
But the second law of thermodynamics teaches us that if you reduce entropy somewhere, you have to pay for it somewhere else. Using this fact, it’s possible to work out the minimum energy needed to capture some amount of carbon dioxide.
Doing the Math on Carbon Capture
The graph below shows the theoretical minimum energy needed to extract 1 tonne of CO₂ from the air, plotted versus the fraction of CO₂ in the air.
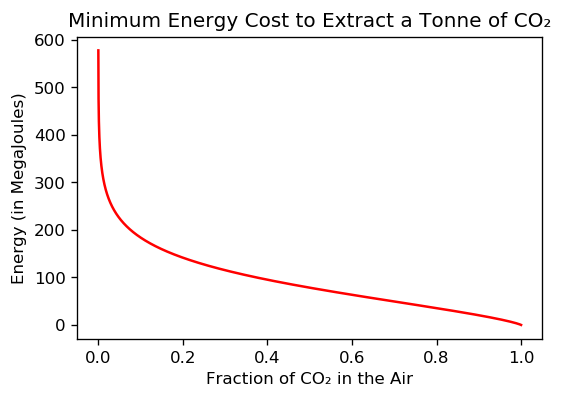
The main takeaway is that as the CO₂ concentration decreases, the cost of extracting it blows up! The concentration of CO₂ in the air is approximately 400 parts per million, or 0.0004 when expressed as a decimal fraction. So we’re very much in the vertical line part of this graph.
Because CO₂ is a trace gas in the atmosphere, extracting carbon from the air is like panning for gold — to extract 1 tonne of CO₂, you need to sift through over 2,000 tonnes of air — and this makes carbon capture unavoidably expensive & inefficient.
Let’s make this graph more useful by switching the horizontal axis to a ‘logarithmic scale’, where each step of the x-axis represents a 10 fold increase in CO₂ concentration.
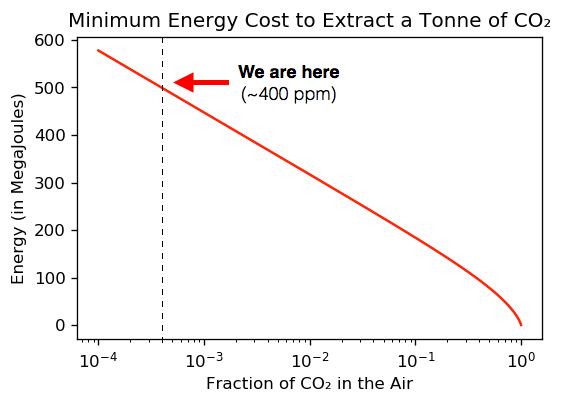
Looking up the current CO₂ concentration in this graph gives us the theoretical minimum cost of extracting CO₂ from the air.
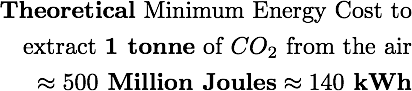
(As a check on the math, this number is within 10% of most published estimates, and within a factor of 2 of other estimates. kWh is short for kiloWatt-hour, which is the standard unit of energy on your electricity bill.)
This number tells us what’s theoretically possible, not what’s technologically feasible. Any real-world machine has unavoidable energy losses which cause it to consume more energy than this.
So how close can we get to this theoretical limit? There’s a lot of debate on this subject, but many estimates suggest the best we can realistically achieve is 10 times the theoretical estimate (aka 10% thermodynamic efficiency).
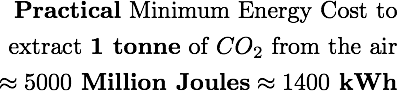
Let’s think about this number in a few ways.
US industries pay ~7 cents for 1 kWh of electricity. Using this as a ballpark conversion rate from energy to money, the practical minimum cost of extracting CO₂ comes out to about ($0.07 / kWh) ⨉ (1,400 kWh / tonne) ≈ $100 per tonne of CO₂.
So physics teaches us two things about the cost of pulling CO₂ from the air.
It can go down somewhat.
It’s still really expensive.
Current estimates place the cost of capturing carbon between $200 and $1,000 per tonne of CO₂. To put that number in context, it would cost the entire US GDP (give or take a factor of 2) to recapture one year’s carbon emissions with existing technology.
The physics suggests there’s room for the cost to go down further, but not by a lot. Even if we optimistically expect a ~2-5 fold drop in the energy cost of carbon capture, recapturing our current annual CO₂ emissions will cost trillions of dollars a year.
And that’s just the energy cost. It doesn’t include the cost of storing the carbon, or the costs of land, infrastructure, maintenance, labor, etc. There’s no getting around it: carbon capture is inherently expensive.
Carbon Capture Machines Need Zero-Carbon Energy
Here’s another way to think about the energy needed for carbon capture technology.
A typical coal power plant emits a tonne of CO₂ for every 1,000 kWh of electricity it generates. Meanwhile, we learned that the energy needed to recapture 1 tonne of CO₂ from the air is at least 1,400 kWh. This means that recapturing the emissions of a coal power plant takes more energy than the coal plant produces!
To put this another way, a carbon capture machine using coal-powered electricity would emit more carbon than it captures. This is why any technology capturing CO₂ from the air must run on zero-carbon energy, or it risks doing more harm than good.
It’s More Efficient to Capture CO₂ at the Source
One last thing. Because the cost of capturing carbon from the air blows up as CO₂ concentration decreases, it takes less energy to capture carbon dioxide at the source of emissions (where it’s concentrated) instead of from the air (where it’s diluted).
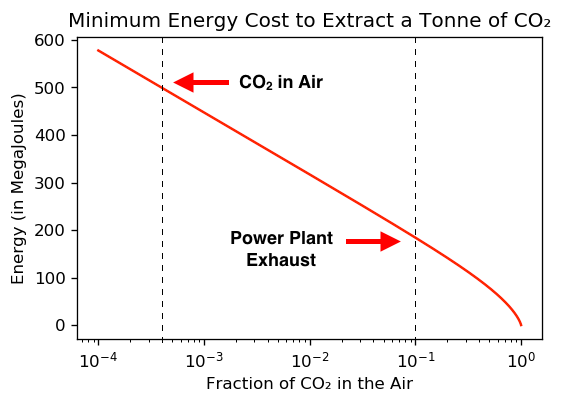
The exhaust gas at a fossil fuel power plant is ~10-15% CO₂. Meanwhile, the CO₂ level in the atmosphere is currently ~0.04%. This gives us a ~2-3 fold reduction in the theoretical minimum cost of capturing CO₂ if we capture it directly at the source.
(This 2-3 fold difference in the theoretical minimum cost of capturing carbon at the source vs. from the air is roughly in agreement with published estimates.)
The Takeaways
Capturing CO₂ from the air is expensive because CO₂ is a dilute gas.
It would currently cost the entire annual US GDP ($20 trillion) to capture 1 year’s global CO₂ emissions.
Even if we (optimistically) expect this cost to drop ~2-5 fold in the future, it’s still expensive.
This is why future carbon capture technologies are no substitute for reducing carbon emissions today. They’ll help us cover the last miles on our path to zero emissions, but they’re too expensive & too inefficient to take us most of the way.
As the authors of a highly-cited 2014 study of carbon capture put it,
“it is highly likely that air capture will offer one of the most expensive options for mitigating climate change. For this reason, other, cheaper options for addressing climate change such as reducing the carbon intensity of electricity generation through efficiency savings in existing power plants, increased deployment of renewable energy technologies, nuclear power and [sequestering carbon] should be aggressively pursued before air capture is considered.”
References
This article builds on an insightful Twitter thread by the climate scientist Andrew Dressler, where he explains how to use physics to estimate the energy needed for direct air carbon capture.

If you’re interested in learning more about entropy, check out my interactive explainer.
Here’s my write-up of the physics behind this article, equations and all.
Here are some in-depth references on the energy limits of carbon capture:
Thanks for reading! If you’d like to see more climate science explainers like this, please consider supporting my writing on Patreon. It helps me keep this newsletter free, and allows me to spend time researching and writing it.